Solid-state battery technology promises a significant leap forward in energy storage. Unlike traditional lithium-ion batteries, these innovative devices utilize solid electrolytes, leading to enhanced safety, higher energy density, and potentially faster charging times. This technology has the potential to revolutionize electric vehicles, portable electronics, and grid storage, pushing the boundaries of what’s possible in power solutions.
The core principles behind solid-state batteries revolve around the unique properties of solid electrolytes. These materials, unlike the liquid electrolytes in lithium-ion batteries, offer improved safety by eliminating the risk of leakage and thermal runaway. This is a crucial aspect for applications like electric vehicles, where safety is paramount.
Introduction to Solid-State Batteries: Solid-state Battery Technology
Solid-state batteries represent a significant advancement in energy storage technology, promising enhanced safety, performance, and potentially, higher energy density compared to their lithium-ion counterparts. These batteries utilize solid electrolytes, eliminating the flammable liquid electrolytes found in conventional lithium-ion batteries. This shift holds the key to unlocking greater energy storage potential and safety improvements, particularly in demanding applications like electric vehicles and portable electronics.Solid-state batteries differ fundamentally from lithium-ion batteries in their electrolyte composition and structure.
While lithium-ion batteries rely on liquid or polymer electrolytes, solid-state batteries employ solid materials to facilitate ion transport. This critical distinction leads to a range of advantages and disadvantages that influence their viability in various applications.
Fundamental Differences from Lithium-ion Batteries
Solid-state batteries differ significantly from conventional lithium-ion batteries. Lithium-ion batteries utilize liquid or polymer electrolytes, while solid-state batteries use solid electrolytes. This fundamental difference impacts safety, performance, and manufacturing processes. Liquid electrolytes are flammable and pose a safety risk, whereas solid electrolytes offer improved safety characteristics. The solid electrolyte’s ionic conductivity also plays a crucial role in determining the battery’s overall performance, which can be influenced by factors such as the type of solid electrolyte used.
Key Advantages and Disadvantages
Solid-state batteries present several advantages over existing battery technologies, including improved safety due to the elimination of flammable liquid electrolytes. They also have the potential for higher energy density, enabling more compact and powerful devices. However, challenges remain, such as achieving sufficient ionic conductivity in the solid electrolyte, which can impact battery performance. The manufacturing process for solid-state batteries can also be more complex than that for lithium-ion batteries, potentially leading to higher production costs.
History of Solid-State Battery Research and Development
Solid-state battery research has been ongoing for decades, with various research groups exploring different solid electrolyte materials. Early efforts focused on understanding the fundamental properties of solid electrolytes and their suitability for battery applications. Recent advances in materials science and manufacturing techniques have led to more promising results, pushing the field closer to commercial viability. Notable milestones include the development of novel solid electrolytes with improved ionic conductivity and the refinement of fabrication processes for solid-state battery cells.
Solid Electrolyte Types
Understanding the different types of solid electrolytes used in solid-state batteries is crucial for appreciating the challenges and opportunities in this field. Different solid electrolytes exhibit varying characteristics, impacting the performance and applications of the resulting battery.
Electrolyte type | Advantages | Disadvantages | Applications |
---|---|---|---|
Ceramic-based (e.g., Li7La3Zr2O12, LLZO) | High thermal stability, good ionic conductivity, and chemical stability. | Relatively low ionic conductivity at room temperature, and high manufacturing cost. | High-power applications, including electric vehicles. |
Polymer-based (e.g., polyethylene oxide (PEO) based electrolytes) | Flexible, potentially lower cost. | Low ionic conductivity, and limited thermal stability. | Flexible electronics, wearable devices. |
Glass-based | High ionic conductivity and potentially high energy density. | Low thermal stability and difficulty in fabrication. | Electric vehicle applications. |
Solid-state inorganic | High safety, potential high energy density | Low ionic conductivity and high manufacturing cost | Potential applications in stationary energy storage. |
Materials Science and Design
Solid-state batteries, promising a leap forward in energy storage, demand meticulous material selection and design. The core components – electrolytes, electrodes, and current collectors – must exhibit exceptional performance characteristics to ensure high energy density, safety, and longevity. This section delves into the key materials employed and the critical challenges in their design.
Key Materials in Solid-State Batteries
The materials chosen for solid-state batteries are paramount to their success. These materials must possess a synergistic interplay of properties to enable high energy density, enhanced safety, and long lifespan. Electrolytes, electrodes, and current collectors form the crucial triad.
- Electrolytes: Solid electrolytes, replacing traditional liquid electrolytes, are crucial for enabling the solid-state architecture. These materials must exhibit high ionic conductivity to facilitate ion transport, enabling efficient charge transfer. A critical property is chemical stability, which prevents unwanted reactions with the electrodes. Examples include ceramic, polymer, and glass-based electrolytes.
- Electrodes: The electrodes, typically composed of lithium metal or lithium-metal oxides, are responsible for storing and releasing charge. The selection of electrode materials must balance electrochemical activity with compatibility with the chosen electrolyte. Factors such as surface area and the presence of active materials greatly influence performance.
- Current Collectors: These materials provide a pathway for electrons to flow from the electrode to the external circuit. The current collectors must exhibit high electrical conductivity and chemical stability to maintain performance over the battery’s lifetime. Common choices include metals like copper and aluminum.
Comparison of Solid Electrolyte Materials
Different solid electrolyte materials offer varying characteristics, each with its own strengths and weaknesses. Choosing the appropriate material is crucial for the overall battery performance.
- Ceramic Electrolytes: These materials often demonstrate high ionic conductivity at elevated temperatures, crucial for high-power applications. However, their ionic conductivity at room temperature is often lower than other types. Ceramic electrolytes are typically characterized by their high chemical stability and resistance to degradation.
- Polymer Electrolytes: These offer the advantage of flexibility and potentially lower processing costs. Their ionic conductivity is typically lower compared to ceramic electrolytes, especially at lower temperatures. Polymer electrolytes are often favored for their flexibility but may exhibit lower chemical stability and mechanical strength.
- Glass-Based Electrolytes: These electrolytes occupy a middle ground, often possessing better ionic conductivity than polymers at room temperature. Their mechanical strength is often higher than polymers, but lower than ceramics. The selection criteria for these materials are influenced by the intended operating temperature range and the desired performance characteristics.
Challenges in Solid Electrolyte Design
Designing stable and high-performance solid electrolytes presents significant challenges.
- Achieving High Ionic Conductivity at Room Temperature: A significant hurdle is achieving sufficiently high ionic conductivity at ambient temperatures. This is essential for practical applications and avoids the need for elevated temperatures during operation.
- Ensuring Chemical Stability: Maintaining the chemical stability of the electrolyte in contact with the electrodes is crucial to prevent undesirable reactions and degradation. This aspect directly impacts the battery’s lifespan.
- Improving Mechanical Properties: Solid electrolytes should possess adequate mechanical strength and stability to endure the stress of repeated charging and discharging cycles. This includes managing the strain and preventing cracking.
Interface Engineering in Solid-State Batteries
The interface between the solid electrolyte and electrodes plays a critical role in determining the battery’s performance.
- Importance of Interface Engineering: Proper interface engineering is essential for achieving optimal performance in solid-state batteries. The interface must facilitate efficient charge transfer and prevent unwanted side reactions, ensuring the stability of the battery’s performance.
Improving Solid-State Battery Electrodes
Optimizing electrode performance is vital for improving the overall battery characteristics.
- Surface Modification: Techniques such as surface coating and doping can be employed to enhance the electrochemical activity and stability of the electrodes. The enhanced surface area also plays a role.
- Nanostructuring: Creating nanostructured electrodes can increase the active surface area and improve electron and ion transport, leading to improved performance.
Summary of Electrode Materials Properties
Material | Conductivity (S/cm) | Stability (Cycles) | Reactivity |
---|---|---|---|
Lithium Metal | High | Moderate | High |
Lithium Nickel Oxide | Moderate | High | Moderate |
Lithium Manganese Oxide | Low | High | Low |
Lithium Cobalt Oxide | Moderate | High | Moderate |
Manufacturing Processes
Current solid-state battery manufacturing processes face significant challenges in achieving cost-effective and scalable production. The intricate nature of these batteries, involving multiple layers of materials with precise control over their properties, demands sophisticated fabrication techniques. These techniques must also be adaptable to accommodate various solid electrolyte materials and ensure the high performance and safety critical for commercialization.The manufacturing processes for solid-state batteries are still evolving, aiming to overcome the hurdles of material compatibility, uniform distribution, and precise control of layer thicknesses.
Different techniques are being explored and optimized to address these challenges, with the ultimate goal of achieving cost-effective and reliable large-scale production.
Current Manufacturing Processes
Various techniques are being employed for fabricating solid-state batteries, each with its own set of advantages and disadvantages. These techniques need to be optimized for specific solid electrolyte materials to ensure proper performance and safety.
Fabrication Techniques
Different fabrication techniques are being investigated for the production of solid-state batteries. These include thin-film deposition, solid-state synthesis, and spray pyrolysis. Each method offers unique capabilities and limitations, influencing the final battery performance.
- Thin-film deposition techniques, such as sputtering and chemical vapor deposition (CVD), enable the precise control of thin layers of materials. This precision is crucial for creating the complex layered structures required in solid-state batteries. However, these methods often require specialized equipment and can be costly, limiting their scalability for mass production. Examples of thin-film deposition applications in other fields include the production of microelectronic components and solar cells.
- Solid-state synthesis involves reacting solid materials to form new compounds. This method is suitable for creating composite materials and homogeneous structures. However, achieving desired stoichiometry and controlling the resulting microstructure can be challenging, especially for complex solid electrolytes. In other industries, solid-state synthesis is commonly used for producing high-purity ceramics and specialized powders.
- Spray pyrolysis is a cost-effective method for producing coatings and thin films. It involves spraying a solution of the desired material onto a substrate, which then undergoes pyrolysis to form the solid material. Spray pyrolysis is relatively easy to scale up compared to some other techniques, but controlling the uniformity of the coating and the final microstructure remains a challenge.
Spray pyrolysis is commonly used in the production of metal oxides and other materials for various applications.
Specific Manufacturing Process Steps (Example: Solid-State Synthesis)
A typical solid-state synthesis process for a solid-state battery involves several key steps:
- Material preparation: High-purity solid materials are weighed and mixed in precise stoichiometric ratios.
- Heating and grinding: The mixed materials are heated in a furnace to drive reactions. Grinding helps achieve a uniform distribution and desired particle size.
- Characterization: The resulting material is characterized using techniques like X-ray diffraction (XRD) and scanning electron microscopy (SEM) to determine its phase composition, particle size, and morphology. This step is essential to understand and control the final material’s properties.
- Electrolyte deposition: The synthesized solid electrolyte material is then deposited onto a suitable substrate, which could be a current collector or separator material. Precise deposition methods are needed to ensure a uniform and continuous layer.
- Electrode fabrication: The active materials for the positive and negative electrodes are mixed with a binder and conductive additive. The resulting paste is then applied to the electrolyte layer. This step is critical for maintaining the structural integrity and electrical conductivity of the electrodes.
- Assembly and testing: The electrodes are assembled with the electrolyte layer, and the battery is tested under various conditions to evaluate its performance and safety. This process involves sealing the components and checking the electrical properties.
Challenges in Scaling Up Production
Scaling up solid-state battery production presents significant challenges, including:
- Material uniformity: Ensuring consistent material properties and composition across large production runs is crucial for reliable battery performance.
- Cost-effectiveness: The high cost of specialized equipment and materials needs to be addressed to make solid-state batteries commercially viable.
- Process optimization: Optimizing the manufacturing process to minimize defects and improve efficiency is essential for scaling up production.
Quality Control
Quality control measures are crucial to ensure the consistency and safety of solid-state batteries. Techniques include:
- Material characterization: Thorough analysis of the materials at different stages of the process is essential to ensure their quality.
- Process monitoring: Real-time monitoring of the manufacturing process parameters helps identify and correct deviations from standard procedures.
- Performance testing: Rigorous testing of the final battery products under various conditions is crucial to evaluate their safety and performance.
Manufacturing Techniques Comparison
Technique | Advantages | Disadvantages | Applications |
---|---|---|---|
Thin-film deposition | Precise control over layer thickness, high purity | Costly equipment, potentially lower throughput | High-performance electronics, specialized sensors |
Solid-state synthesis | Homogeneous material composition, suitable for complex structures | Time-consuming, potential for defects | Ceramic materials, advanced battery components |
Spray pyrolysis | Cost-effective, relatively scalable | Controlling uniformity can be challenging, potential for contamination | Coatings, thin films for diverse applications |
Performance Characteristics
Solid-state batteries (SSBs) are poised to revolutionize energy storage, promising significant improvements over conventional lithium-ion batteries (LIBs) in terms of performance and safety. Key performance characteristics, including energy density, power density, safety, and cycle life, are crucial factors determining their viability and widespread adoption. Understanding these characteristics and the factors influencing them is vital for successful SSB development.
Energy Density
Energy density, a measure of the energy stored per unit volume or mass, is a critical aspect of battery performance. SSBs are expected to achieve comparable or potentially higher energy densities than LIBs. This depends largely on the solid electrolyte material used and the electrode design. The use of high-capacity materials in the electrodes is a key driver in enhancing energy density.
For instance, the utilization of lithium metal as an anode material, while posing safety challenges, could lead to significant increases in energy density.
Power Density
Power density, representing the rate at which energy can be delivered, is another important factor. While theoretical predictions suggest SSBs could exhibit high power densities, practical implementation faces challenges related to the ionic conductivity of the solid electrolyte. The ionic conductivity of the solid electrolyte material directly impacts the battery’s ability to rapidly deliver power. Researchers are actively investigating new solid electrolyte materials with improved ionic conductivity to overcome this limitation.
Safety
Safety is paramount in battery technology. SSBs offer the potential for enhanced safety due to the elimination of flammable organic electrolytes. However, the presence of solid electrolytes, while safer than liquid electrolytes, can still present challenges. These include issues related to mechanical stability and the potential for thermal runaway. Solid electrolytes are generally more resistant to short circuits and leakage compared to liquid electrolytes.
Careful material selection and robust cell design are essential for maximizing safety.
Cycle Life
Cycle life, the number of charge-discharge cycles a battery can endure before its performance degrades significantly, is a key factor in determining the long-term viability of the technology. The electrochemical stability of the materials used in SSBs plays a significant role in determining cycle life. The stability of the solid electrolyte and the electrode materials against degradation during repeated charge-discharge cycles is a critical aspect.
SSBs are expected to demonstrate a comparable or improved cycle life compared to LIBs, but this remains a focus of ongoing research and development.
Impact of Temperature, Solid-state battery technology
Temperature significantly impacts the performance of SSBs. The ionic conductivity of the solid electrolyte material varies with temperature. Increased temperature typically leads to improved ionic conductivity, enabling faster charge-discharge rates and higher power densities. However, extreme temperatures can also lead to degradation of the materials, potentially impacting cycle life and safety. The operating temperature range of SSBs is crucial for applications requiring reliable performance across diverse environments.
Factors Influencing Safety
The safety of SSBs is contingent upon several factors. These include the inherent stability of the solid electrolyte material, the mechanical integrity of the cell design, and the thermal management system. The solid electrolyte should possess high mechanical strength to prevent leakage and ensure structural integrity. A robust thermal management system is essential to prevent overheating and thermal runaway, a critical safety concern.
Additionally, the design of the battery cell itself needs to consider potential hazards.
Electrochemical Stability
Electrochemical stability is critical for the long-term performance of SSBs. The solid electrolyte and electrode materials must exhibit high stability against electrochemical reactions and degradation during repeated charge-discharge cycles. Electrochemical stability is essential to maintain the battery’s performance and capacity over time. The ability of the materials to withstand the electrochemical stresses of repeated charging and discharging without significant degradation is a key determinant of cycle life.
Comparison with Lithium-Ion Batteries
SSBs show promise for improving on several aspects of conventional LIBs. They are expected to offer higher energy densities, improved safety profiles, and potentially longer cycle lives. However, challenges remain in achieving sufficient power density and developing cost-effective manufacturing processes. The performance metrics vary significantly based on the specific materials used in the solid-state battery design.
Performance Metrics Table
Solid-State Battery Type | Energy Density (Wh/kg) | Power Density (W/kg) | Cycle Life (Cycles) |
---|---|---|---|
Lithium-sulfur (Li-S) | ~1000 | ~500 | ~500 |
Lithium-metal oxide (Li-MO) | ~700 | ~400 | ~800 |
All-solid-state (ASS) | ~600 | ~300 | ~1000 |
Note: Values are approximate and can vary significantly depending on the specific material and cell design.
Applications and Market Potential
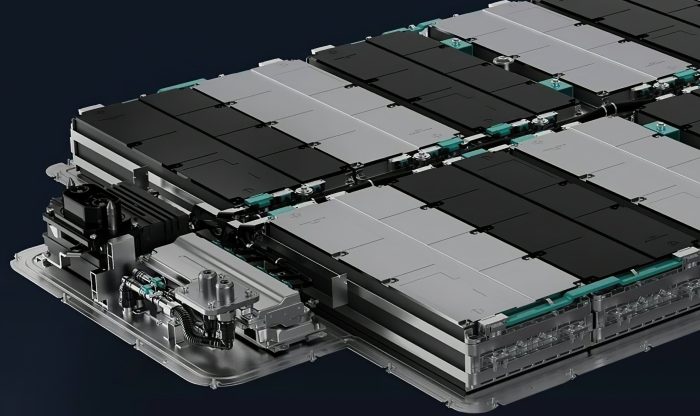
Solid-state batteries (SSBs) hold immense promise for revolutionizing various sectors, particularly those heavily reliant on energy storage, such as electric vehicles (EVs) and portable electronics. Their unique characteristics, including higher energy density, enhanced safety, and potentially longer lifespans, could dramatically impact the future of these industries. This section delves into the potential applications of SSBs, the market demand they might generate, and the current status of their commercialization efforts.
Potential Applications
Solid-state batteries are poised to disrupt several key sectors. Their superior properties, including higher energy density and enhanced safety features, offer significant advantages over existing lithium-ion battery technology. This translates to greater range for electric vehicles, faster charging times for portable electronics, and improved grid stability.
- Electric Vehicles (EVs): SSBs could significantly enhance the driving range and performance of EVs. Increased energy density allows for larger battery packs in the same physical space, potentially leading to longer driving ranges without compromising vehicle weight. This is crucial for mass adoption of EVs and expanding their market share.
- Portable Electronics: Smaller, lighter, and more powerful batteries for smartphones, laptops, and other portable devices are a direct result of SSB technology. The increased energy density, coupled with improved safety features, could lead to substantial advancements in portable device performance and usability.
- Grid Storage: SSBs offer the potential to enhance grid stability and reliability. Their long lifespan and high energy density make them ideal for large-scale energy storage solutions, enabling integration of renewable energy sources like solar and wind power into the grid more efficiently. This will address the intermittency challenges associated with these renewable energy sources.
Market Demand and Potential
The market potential for solid-state batteries is substantial, driven by increasing demand for electric vehicles, advancements in portable electronics, and the growing need for reliable grid storage solutions. Forecasting precise market sizes is challenging due to the technology’s current stage of development, but the potential for significant growth is undeniable.
- Electric Vehicle Market: The global EV market is rapidly expanding, fueled by government incentives and consumer interest. SSBs are expected to play a crucial role in supporting this growth by delivering the range and performance needed to meet consumer expectations. Companies like Tesla and Volkswagen are actively investing in SSB research, highlighting the industry’s anticipation for their potential.
- Portable Electronics Market: The constant demand for smaller, more powerful portable devices drives the need for superior battery technology. SSBs have the potential to provide significant improvements in battery performance, leading to enhanced user experiences and greater adoption rates for new devices.
- Grid Storage Market: Integrating renewable energy sources like solar and wind into the electricity grid requires advanced energy storage solutions. SSBs offer a promising path toward achieving this, enabling grid stability and reliability.
Commercialization Status
Currently, solid-state battery technology is still in its early stages of commercialization. While several companies and research institutions are actively developing and testing different SSB chemistries, widespread adoption is not yet imminent. Overcoming technical challenges related to manufacturing scalability and cost-effectiveness is crucial for achieving wider market penetration.
- Challenges to Commercialization: Challenges remain in the production and scaling of SSB technology. Manufacturing processes are still under development, and significant investment is required to achieve cost-effectiveness. Safety testing and long-term durability assessments are also essential for widespread adoption.
- Examples of Companies and Research Institutions: Numerous companies and research institutions are actively involved in solid-state battery development, including, but not limited to, Sony, Panasonic, and research teams at leading universities. These efforts underscore the significance and potential impact of this technology.
Potential Applications Table
Application | Expected Benefits | Associated Challenges | Commercialization Status |
---|---|---|---|
Electric Vehicles | Increased range, faster charging, improved safety | Manufacturing scalability, cost-effectiveness, long-term durability | Early stage, but significant investment |
Portable Electronics | Smaller, lighter, longer-lasting batteries | Battery integration, manufacturing cost, safety standards | Limited commercialization, but promising research |
Grid Storage | Improved grid stability, enhanced reliability, integration of renewable energy | Large-scale manufacturing, cost-effectiveness, battery longevity | Research focused on large-scale storage solutions |
Safety Considerations
Solid-state batteries, while promising, present unique safety challenges compared to lithium-ion batteries. Understanding these risks and implementing robust safety measures are crucial for their widespread adoption. Thorough analysis of potential hazards and development of effective mitigation strategies are essential for ensuring the safe operation of solid-state battery systems.Thermal runaway, a rapid and uncontrolled exothermic reaction, is a significant concern in all battery chemistries, and solid-state batteries are not exempt.
The mechanisms involved and their specific characteristics in solid-state batteries require careful investigation and preventative measures to minimize the risk of fire or explosion. Safety protocols must be adapted to the unique properties of solid-state electrolytes and electrode materials to effectively assess potential risks.
Thermal Runaway Mechanisms
Solid-state batteries differ from lithium-ion batteries in their thermal runaway mechanisms due to their unique materials and construction. Solid-state electrolytes, with their differing thermal properties and reaction kinetics, can contribute to unique failure modes. The presence of solid components and their interactions with the electrodes lead to different reaction pathways compared to lithium-ion systems, which primarily involve liquid electrolytes.
Different degradation modes can result in differing failure characteristics.
Safety Testing Protocols
Rigorous safety testing protocols are crucial for evaluating the performance and reliability of solid-state batteries under various stress conditions. These protocols typically involve subjecting the batteries to high-temperature and high-current conditions to identify potential failure modes. Tests simulating real-world scenarios, such as short circuits and mechanical abuse, are also essential. Specialized equipment and methodologies, adapted to the unique properties of solid-state batteries, are essential.
For instance, high-temperature thermal cycling and accelerated degradation testing are employed to assess long-term stability and safety.
Safety Features in Solid-State Battery Designs
Safety features incorporated into solid-state battery designs are vital for preventing accidents. These include passive safety features, like robust packaging materials and thermal management systems, and active safety mechanisms, like advanced thermal sensors and sophisticated shut-off mechanisms. Effective design strategies and material selection play a crucial role in reducing the risk of thermal runaway and preventing hazardous events.
Examples of passive features include thermal insulators and robust containment systems, while active features include automatic cut-off circuits and active cooling systems.
Solid-state batteries are poised for significant advancements, promising higher energy density and safety. AI trends in 2025, like those highlighted in this insightful piece on AI trends 2025 , are expected to play a crucial role in optimizing battery performance and material science research. This could lead to more efficient and reliable solid-state battery technology in the near future.
Fire and Explosion Prevention Measures
Proactive measures for fire and explosion prevention are essential for the safe operation of solid-state batteries. These include stringent quality control during manufacturing, careful material selection, and thorough testing to identify and mitigate potential risks. Proper handling and storage procedures, as well as emergency response plans, are critical aspects of a comprehensive safety program. Emergency protocols, including fire suppression systems and personnel training, should be in place to handle any potential incidents.
Compliance with industry safety standards and regulations is critical to minimize risks.
Challenges and Future Directions
Solid-state batteries (SSBs) promise significant advancements in energy storage, but their widespread adoption faces numerous hurdles. These challenges stem from the intricate interplay of materials science, manufacturing processes, and performance characteristics. Overcoming these obstacles is crucial for realizing the full potential of SSBs and their transformative impact on various sectors.
Key Challenges in SSB Development
The transition from lithium-ion batteries to SSBs is not straightforward. Significant technological hurdles exist, hindering commercialization. These include the need for highly conductive and stable solid electrolytes, compatible electrode materials, and robust manufacturing processes. The inherent complexities of these interconnected factors demand a multifaceted approach to overcoming these challenges.
Technological Hurdles
Several key technological hurdles hinder the development and commercialization of solid-state batteries. These obstacles range from material incompatibility to manufacturing limitations. Addressing these hurdles requires innovative research and development strategies.
- Solid Electrolyte Challenges: Solid electrolytes, crucial for ionic conductivity, often exhibit poor compatibility with electrode materials, leading to interfacial instability and reduced performance. This incompatibility results in significant performance degradation over time. Furthermore, achieving high ionic conductivity at room temperature remains a critical challenge. For instance, certain solid electrolytes demonstrate excellent ionic conductivity at high temperatures, but their performance drops considerably at lower temperatures, making them unsuitable for everyday applications.
- Electrode Material Compatibility: Designing electrode materials that effectively interact with the solid electrolyte without causing degradation or hindering charge transfer is a major hurdle. The different chemical properties of the solid electrolyte and the electrode materials often lead to interfacial issues. For example, some solid electrolytes may react with certain electrode materials, forming undesirable compounds that compromise battery performance.
- Manufacturing Scalability: Developing cost-effective and scalable manufacturing processes for solid-state batteries is essential for commercial viability. Current manufacturing techniques often lack the efficiency and precision required for mass production. This is particularly true for the precise deposition of thin solid electrolyte layers, critical for battery performance.
Research Directions to Address Challenges
Addressing these challenges requires a multi-pronged research approach. Focus areas include novel materials synthesis, improved manufacturing processes, and enhanced performance characterization.
- Developing Novel Solid Electrolytes: Research into novel solid electrolytes with high ionic conductivity at room temperature, combined with enhanced compatibility with electrode materials, is crucial. Exploring materials like garnet-type electrolytes, or developing composite solid electrolytes with enhanced ionic conductivity, are key areas of focus.
- Optimizing Electrode-Electrolyte Interfaces: Developing surface treatments and coatings to improve the interfacial compatibility between the electrode materials and the solid electrolyte is essential. Strategies like atomic layer deposition or advanced surface modification techniques can improve interface stability and reduce degradation.
- Advancements in Manufacturing Techniques: Research into innovative manufacturing processes for large-scale production, such as roll-to-roll techniques, can significantly reduce production costs and increase throughput. Developing new methods for precise deposition and integration of solid electrolyte layers into the battery structure is also crucial.
Potential Solutions
Challenge | Potential Solution | Example | Impact |
---|---|---|---|
Poor electrolyte-electrode compatibility | Developing surface treatments or coatings to improve interfacial stability | Atomic layer deposition of a protective layer on the electrode surface | Improved cycle life and performance |
Low ionic conductivity at room temperature | Synthesizing new solid electrolytes with enhanced ionic conductivity at lower temperatures | Development of new garnet-type solid electrolytes | Improved performance at practical temperatures |
Scalability of manufacturing | Exploring roll-to-roll manufacturing techniques for solid electrolyte deposition | Adapting existing flexible electronics manufacturing methods | Reduced production costs and increased throughput |
Comparison with Other Battery Technologies
Solid-state batteries represent a significant advancement in battery technology, but they are not the only contenders in the field. Understanding their position relative to other emerging technologies is crucial for assessing their potential and limitations. This section will compare solid-state batteries to other promising battery types, highlighting their respective advantages and disadvantages.
Key Performance Characteristics Comparison
Various battery technologies exhibit unique characteristics in terms of energy density, power density, safety, and cost. A comparative analysis is essential to evaluate the suitability of each technology for different applications.
Battery Technology | Energy Density (Wh/kg) | Power Density (W/kg) | Safety |
---|---|---|---|
Solid-State Batteries | Potentially high, exceeding 500 Wh/kg in some prototypes | High, potentially exceeding 10 kW/kg | Generally high, due to the solid electrolyte |
Lithium-ion Batteries (Li-ion) | High, typically ranging from 100 to 250 Wh/kg | High, typically ranging from 1 to 5 kW/kg | Relatively high, but safety concerns exist (thermal runaway) |
Lithium-sulfur Batteries (Li-S) | Potentially very high, exceeding 1000 Wh/kg in theory | Moderate, with challenges in achieving high power density | Generally lower than Li-ion, due to the sulfur cathode |
Lithium-air Batteries (Li-air) | Potentially very high, theoretically exceeding 11,000 Wh/kg | Moderate to low, depending on the design | Moderate, but with significant challenges in long-term stability |
Advantages and Disadvantages of Each Technology
Each battery technology possesses unique advantages and disadvantages, influencing their suitability for various applications.
- Solid-State Batteries: Their solid electrolytes offer enhanced safety compared to liquid electrolytes in lithium-ion batteries. This, combined with the potential for high energy and power densities, makes them attractive for electric vehicles (EVs) and other high-demand applications. However, current manufacturing processes are less developed than for Li-ion batteries, resulting in higher costs. Furthermore, challenges remain in achieving long-term stability and scalability.
- Lithium-ion Batteries: Li-ion batteries are currently the dominant technology, boasting high energy and power densities and established manufacturing processes. Their widespread adoption in consumer electronics and EVs has made them highly cost-effective. However, safety concerns, such as thermal runaway, are inherent in the use of liquid electrolytes.
- Lithium-sulfur Batteries: Li-S batteries have the potential for very high energy density, exceeding Li-ion batteries, due to the high theoretical capacity of sulfur. However, challenges in sulfur cathode design and electrolyte stability limit their performance. The current development is focused on improving the cycle life and overcoming these issues.
- Lithium-air Batteries: Li-air batteries possess the highest theoretical energy density of all these technologies. This theoretical advantage stems from the high reactivity of air. However, practical challenges in achieving stable and long-lasting operation, coupled with the need for complex components, limit their current viability.
Specific Strengths of Solid-State Batteries
Solid-state batteries excel in several key areas compared to other technologies. Their solid electrolytes enhance safety, mitigating the risk of short circuits and thermal runaway. This safety aspect is particularly crucial for applications requiring high power density, such as electric vehicles. The potential for higher energy density, coupled with good power density, positions solid-state batteries to disrupt existing markets.
Solid-state battery technology is rapidly advancing, promising significant improvements in energy storage. Analyzing massive datasets, like those generated by testing these batteries, requires robust cloud computing platforms. Tools from Cloud Computing & Big Data are proving crucial for accelerating research and development in this area, ultimately leading to more efficient and reliable solid-state battery production.
Their potential for higher operating voltage also offers advantages over conventional battery types.
Economic and Environmental Impact
Solid-state batteries promise a significant leap forward in battery technology, but their economic viability and environmental footprint are crucial considerations. Their potential to revolutionize various sectors, from electric vehicles to portable electronics, hinges on their ability to compete with existing technologies and minimize their environmental impact. This section explores the economic and environmental implications of this emerging technology.
Potential Economic Impact
Solid-state batteries’ potential to revolutionize the energy storage industry holds significant economic implications. The development and widespread adoption of this technology could lead to substantial investment in research, manufacturing, and infrastructure. Companies involved in battery production, automotive manufacturing, and related industries stand to gain substantial market share. The improved energy density and safety features of solid-state batteries could drive innovation in various applications, opening new avenues for economic growth and job creation.
The shift towards electric vehicles, powered by solid-state batteries, would stimulate demand for battery components and related technologies.
Environmental Impact Reduction
Solid-state batteries offer several avenues for reducing the environmental impact of existing battery technologies. Their superior energy density allows for smaller battery packs in electric vehicles, reducing the overall material consumption and the associated environmental impact. The use of sustainable materials in solid-state battery production, if feasible, will further contribute to a lower environmental footprint. Furthermore, the reduced reliance on rare earth minerals for solid-state battery production, if achievable, would be a major step forward.
Finally, the increased energy efficiency of electric vehicles powered by solid-state batteries translates to lower greenhouse gas emissions.
Cost-Effectiveness of Production
The cost-effectiveness of solid-state battery production remains a key factor in their commercial viability. Current research and development efforts focus on reducing the cost of manufacturing, optimizing material usage, and streamlining production processes. The successful implementation of these strategies is essential for solid-state batteries to compete economically with conventional battery technologies. Pilot production runs and scaling up production facilities are critical steps in making solid-state batteries more cost-competitive.
The initial high costs of production are expected to decrease as economies of scale are realized.
Comparative Analysis of Battery Types
Battery Type | Economic Implications | Environmental Implications | Cost-Effectiveness (Estimate) |
---|---|---|---|
Lithium-ion | Mature market, established supply chain, cost-effective for current applications. | Significant environmental impact due to mining of rare earth elements, manufacturing processes, and end-of-life disposal. | High cost-effectiveness due to mature production processes and large-scale production. |
Solid-state | Potential for significant economic growth in related industries, reduced reliance on specific raw materials. | Potential for reduced environmental impact with the use of sustainable materials, lower energy consumption during operation. | Variable, depending on the material and production process. Initially higher than lithium-ion, but projected to decrease with economies of scale. |
Lead-acid | Cost-effective for some applications, but limited by energy density. | Environmental concerns due to lead pollution in manufacturing and disposal. | Very cost-effective for applications where energy density is not a major concern. |
Note: Cost-effectiveness estimates are based on current data and projections. The actual cost-effectiveness may vary depending on specific technological advancements and market conditions.
Societal Implications
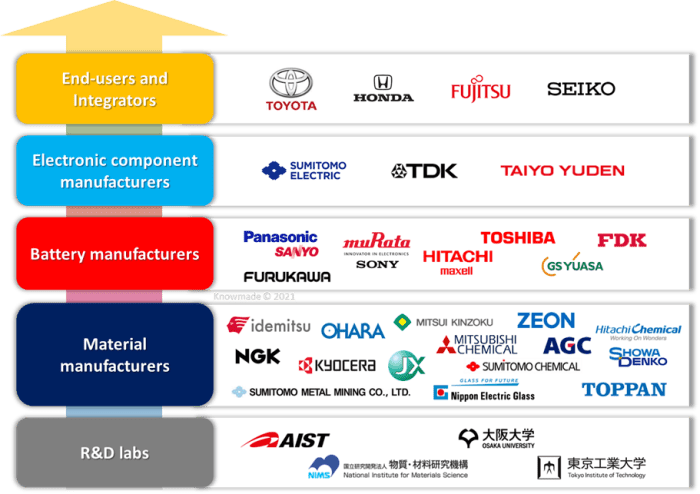
Solid-state batteries, with their potential for higher energy density, longer lifespan, and enhanced safety, hold significant implications for various sectors of society. Their widespread adoption could revolutionize transportation, energy storage, and countless other applications, fostering a more sustainable and technologically advanced future.The transition to solid-state batteries promises transformative changes in various industries. From electric vehicles and grid-scale energy storage to portable electronics and industrial applications, the technology offers the potential for increased efficiency, reduced environmental impact, and economic growth.
This shift will require substantial investment in research, development, and infrastructure, creating new jobs and opportunities.
Impact on Transportation
The automotive industry stands to benefit greatly from solid-state batteries. Improved range, faster charging times, and enhanced safety features will likely drive significant adoption of electric vehicles. This shift could significantly reduce reliance on fossil fuels, leading to lower emissions and a cleaner environment. Further, the reduced weight of solid-state batteries will likely translate to improved vehicle performance.
Impact on Energy Storage
Solid-state batteries are poised to revolutionize energy storage solutions for homes and communities. Their high energy density and long lifespan can enable more efficient grid management, facilitating the integration of renewable energy sources. This improved energy storage could lessen the impact of intermittent energy production, such as solar and wind power, which is crucial for a sustainable energy future.
Impact on Portable Electronics
In the realm of portable electronics, solid-state batteries will likely lead to significant advancements. Enhanced performance, smaller form factors, and extended battery life will result in more powerful and convenient devices. This progress could greatly impact consumers by offering improved functionality in their everyday devices.
Impact on Industrial Applications
Beyond the consumer market, solid-state batteries can find applications in industrial sectors like robotics, manufacturing, and medical devices. Their improved characteristics could drive innovation and automation across various industries.
Role in Sustainable Energy Transition
Solid-state batteries play a critical role in the global transition to a sustainable energy future. Their enhanced energy density and lifespan enable greater efficiency in storing and utilizing renewable energy sources, such as solar and wind power. This facilitates a shift away from fossil fuels, which are major contributors to climate change.
Potential Societal Benefits and Challenges
Societal Benefit | Detailed Description | Societal Challenge | Detailed Description |
---|---|---|---|
Enhanced Energy Independence | Reduced reliance on imported fossil fuels, potentially boosting national energy security. | High Initial Costs | The initial investment required for research, development, and manufacturing could be substantial, potentially hindering widespread adoption in the short term. |
Improved Environmental Outcomes | Reduced carbon emissions from transportation and energy storage, contributing to a cleaner environment. | Material Scarcity | The production of solid-state batteries may rely on specific materials that are scarce or geographically concentrated, raising concerns about resource availability and potential price volatility. |
Economic Growth | Creation of new jobs and industries associated with research, manufacturing, and deployment of solid-state batteries. | Safety Concerns | Addressing potential safety issues related to the new materials and designs of solid-state batteries is crucial for consumer acceptance. |
Increased Efficiency | Higher energy density and longer lifespan lead to more efficient energy storage and usage. | Technological Maturity | Overcoming technical challenges associated with scaling up production and ensuring reliable performance at large-scale deployment is important. |
Final Summary
In conclusion, solid-state battery technology presents a compelling alternative to current battery technology. While challenges remain in manufacturing and scaling up production, the potential benefits in terms of safety, performance, and environmental impact are substantial. Further research and development in materials science and manufacturing processes are key to unlocking the full potential of this groundbreaking technology.
Essential Questionnaire
What are the main differences between solid-state and lithium-ion batteries?
Solid-state batteries use solid electrolytes, unlike lithium-ion batteries which use liquid electrolytes. This difference leads to improved safety and potentially higher energy density in solid-state batteries. However, solid-state batteries currently face challenges in manufacturing scalability and cost.
What are the key safety advantages of solid-state batteries?
The solid electrolyte in solid-state batteries eliminates the risk of leakage and thermal runaway, which are significant safety concerns with liquid electrolyte batteries. This makes them potentially safer for applications like electric vehicles and portable electronics.
What are the major challenges in scaling up solid-state battery production?
Currently, challenges exist in manufacturing processes, particularly in achieving consistent quality and reducing costs at scale. This includes issues in manufacturing the solid electrolytes and integrating them into battery cells.
What are some potential applications of solid-state batteries beyond electric vehicles?
Solid-state batteries could revolutionize various sectors, including portable electronics (like smartphones and laptops) and grid-scale energy storage. Their improved safety and performance characteristics make them attractive for diverse applications.